SPERM WHALES BEACH IN THE NORTH SEA
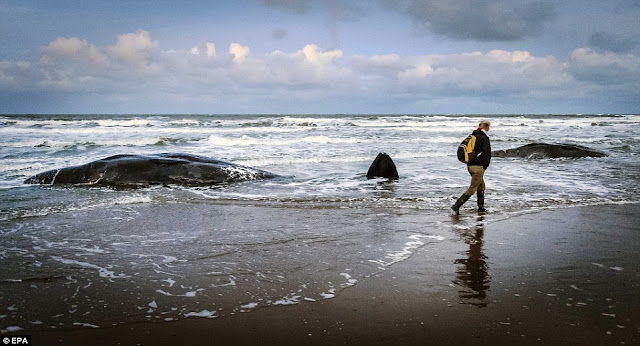
29 Sperm Whales Washed Ashore by Strong Wind-Driven Currents Introduction Whale scientists said, "The animals inadvertently consume plastic and plastic waste, which causes them to suffer and starve with full stomachs." ( link ) They blame the stranding on the garbage floating on the oceans. Think about it! How can a pod of majestic sperm whales using the most advanced biosonar system in the world to locate the squid they eat in the total darkness 5,000 meters below the surface might "inadvertently" identify plastic and ropes and other garbage as food? The truth is that these sperm whales had been suffering biosonar failure ever since they were injured by rapid and excessive pressure changes above the epicenter of an undersea earthquake (aka. seaquake). The injury is barotraumatic in nature and mainly affects the enclosed cranial air spaces. Seaquakes are sudden disturbances in hydrostatic pressure caused by an underwater volcanic explosion o